May 21, 2025
The Shadow of an Electron
Quantum processors: Influencing the trace of ‘missing electrons’ in spin qubits
Amid the race to develop and market practical quantum computers, researchers from the Katsaros group at ISTA pay particular attention to the intriguing physics of special qubits generated in the semiconductor germanium. By harnessing the response of these so-called “hole spin qubits” to magnetic and electric fields, they answer fundamental questions about the physics that could help advance quantum processors. The findings were published in Nature Communications.
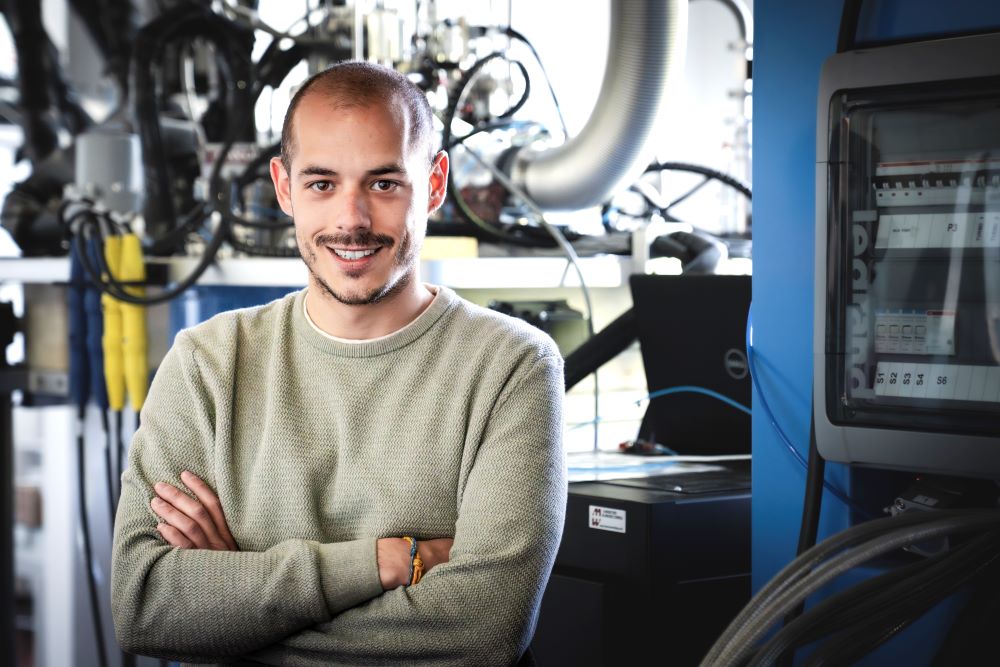
According to a recent TIME article, there is little doubt that the quantum era has begun. Yet, where we stand in the innovation trajectory toward practical quantum computing remains subject to debate. We are confident that quantum computers will one day outperform classical computers in solving specific problems. However, achieving this goal is all but straightforward. In a global race to find the most promising path to this technological breakthrough, myriad research endeavors attempt to advance the most diverse facets of quantum science and technology.
The group of Georgios Katsaros at the Institute of Science and Technology Austria (ISTA) is particularly interested in one material relevant for quantum processors. In contrast to other materials whose native charge carriers are electrons, the semiconductor germanium carries electrical charge with the help of ‘missing electrons,’ called “holes.”
Holes act like positively charged particles that help carry the current. “Germanium is one of the most commonly used semiconductors. It is mainly known for being used in 1947 in the first working transistor. It shows beautiful physics that is still not understood after so many decades,” says Katsaros. But how can tuning the properties of missing electrons in germanium help advance quantum computing?
Now, experimental and theoretical physicists and material scientists around Katsaros and ISTA graduate student Jaime Saez-Mollejo have answered fundamental questions on germanium holes. Their findings could ultimately help advance quantum processors.
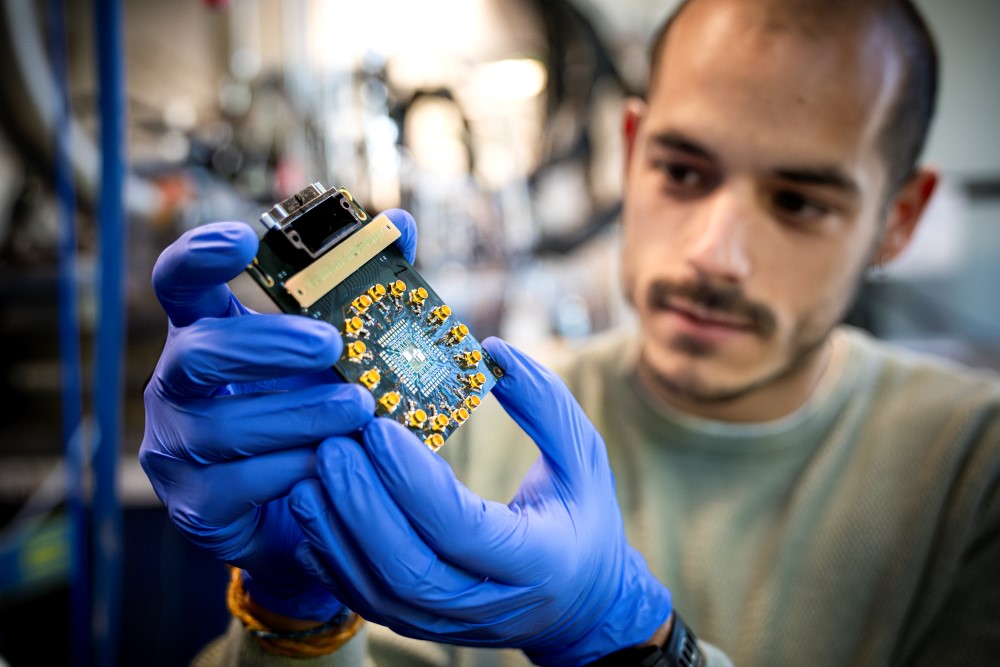
When electrons leave magnetic ‘shadows’
Electrons are inherent to all atoms surrounding us. When an electron leaves its orbital around an atom’s nucleus, its absence creates a net positive charge in the material. Such holes can be deliberately engineered in semiconductor materials like germanium. Like electrons, holes can also move around the material under the effect of electrical fields. It is precisely these properties of germanium that make it useful in quantum technology.
The ‘missing electrons’ conserve their magnetic properties—called “spins”—which point in a given direction, like magnetic ‘shadows’ of the non-existing electrons. These “hole spins” are valuable entities that could act as qubits—the fundamental building blocks of quantum systems named in analogy to the ‘bits’ of classical computation. Thus, holes allow germanium to generate the so-called “hole spin qubits”, special qubits that are the emerging workhorse of semiconducting quantum processors. But the complexity of hole spin qubits does not stop there.
Two dots, one qubit
Whether in electrons or holes, two spins are said to be isolated when they are far apart. Conversely, bringing them into proximity in the material allows them to interact and exhibit quantum properties.
“In our work, we tackled a fundamental question: How to align—and thus control—two interacting hole spins in germanium,” says Saez-Mollejo, the study’s lead author. He and the team examined the parameters that allowed them to tune two spins using magnetic and electric fields.
To this end, they fabricated a chip in collaboration with material scientists at the Polytechnic University of Milan. This chip helped them channel two spins to specific locations on the thin germanium layer, close enough to interact. The locations that confine the holes measure only a few tens of nanometers—comparable to the dimensions of molecules—and are therefore called quantum dots. Having two of them makes the chip a double quantum dot device. Together, the two quantum dots work as a qubit made of two ‘shadows’ of missing electrons.
“The amount of time the qubit stores quantum information depends on how the hole spins are tuned and aligned. This is called the ‘coherence time’. Finding the parameters that influence the spins’ alignment will help us better control the quantum states in the qubits. Ultimately, this is what will allow them to perform quantum computation,” says Saez-Mollejo.
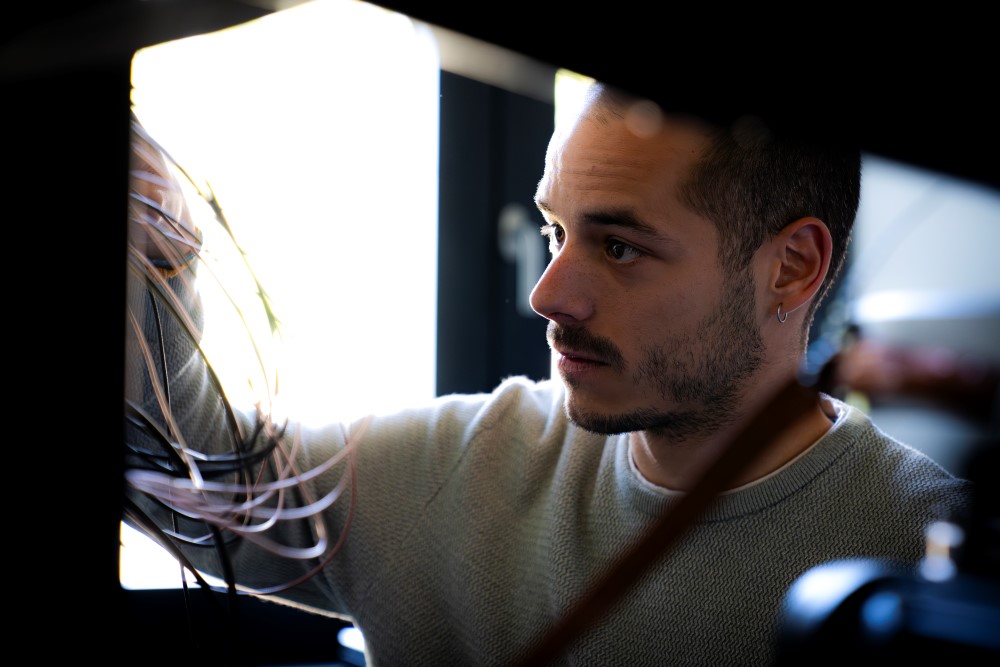
Distinct responses to magnetic and electric fields
When a magnetic field points in the same direction as the thin, planar germanium, it is said to be in-plane with the material. On the other hand, the field is said to be out-of-plane when it is perpendicular to the germanium layer.
With the help of mathematical models, numerical simulations, and experiments, the authors shed light on how the spins’ quantum states interact under various conditions. “We found that the spins align really well when the magnetic field is out of plane,” says Saez-Mollejo. “But when the magnetic field is in plane with the germanium, the spins are misaligned by 45 degrees.”
More strikingly, by dissecting the effects of the electric and magnetic fields on the spins, they found that the spins reacted to them distinctly. While the spin’s misalignment depended on the direction of the magnetic field, it also depended on both the strength and direction of the electric field. On the other hand, with the help of theorists at QuTech, Delft University of Technology, the team also provided the protocol to quantify the misalignment of spins under each condition.
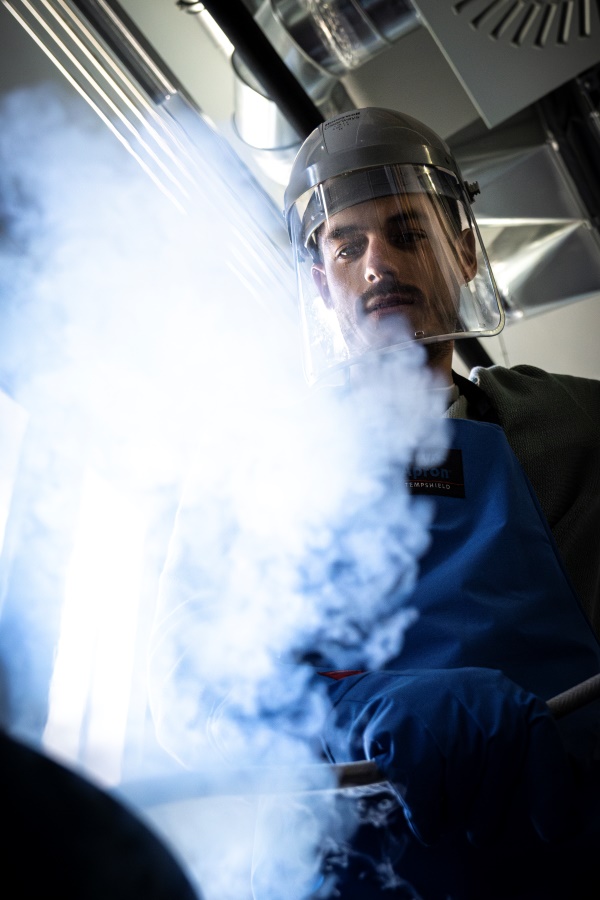
A step in the direction of quantum processors
The present findings on semiconductor spin qubits address fundamental questions on the feasibility of future quantum processors. Unlike electron spins, hole spin qubits have physical properties that allow the fabrication of chips without on-chip micromagnets. Such simplified fabrication requirements would make it possible to scale up the qubit number on processor chips. Thus, understanding how hole spins behave in germanium double quantum dot devices could provide a basis for designing future semiconducting quantum processors.
“Our work might be a little step forward in the full development of quantum processors. Considering the breadth of the quantum computing field, I like to think of our contribution as a little grain of sand that could make an impact,” concludes Saez-Mollejo.
The present research was carried out in collaboration with material scientists at the Polytechnic University of Milan, Italy, and theorists at QuTech, Delft University of Technology, the Netherlands.
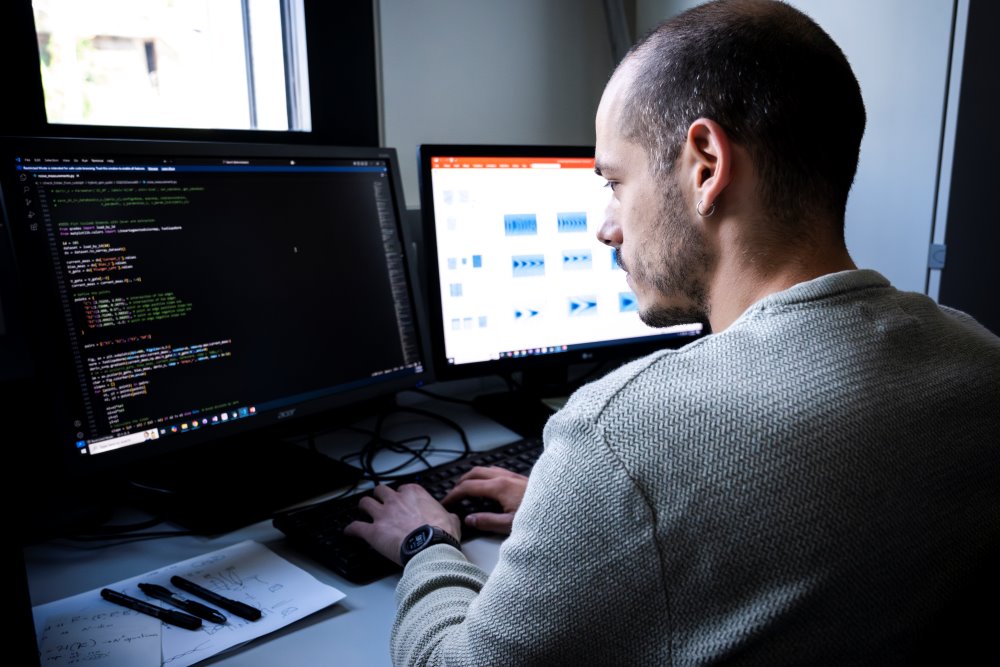
Publication:
Jaime Saez-Mollejo, Daniel Jirovec, Yona Schell, Josip Kukucka, Stefano Calcaterra, Daniel Chrastina, Giovanni Isella, Maximilian Rimbach-Russ, Stefano Bosco & Georgios Katsaros. 2025. Exchange anisotropies in microwave-driven singlet-triplet qubits. Nat Communications. DOI: 10.1038/s41467-025-58969-y
Funding information:
This project was supported by funding from the NOMIS Foundation, the HORIZON-RIA 101069515 project, the FWF Projects with DOI:10.55776/F86 and DOI:10.55776/I5060, the Netherlands Organization of Scientific Research (NWO) under Veni grant VI.Veni.212.223, and the Army Research Office under Award Number: W911NF-23-1-0110.