March 16, 2021
Embryonic tissue undergoes phase transition
Scientists explain a crucial phase of embryonic development in zebrafish with the help of mathematical and physical concepts.
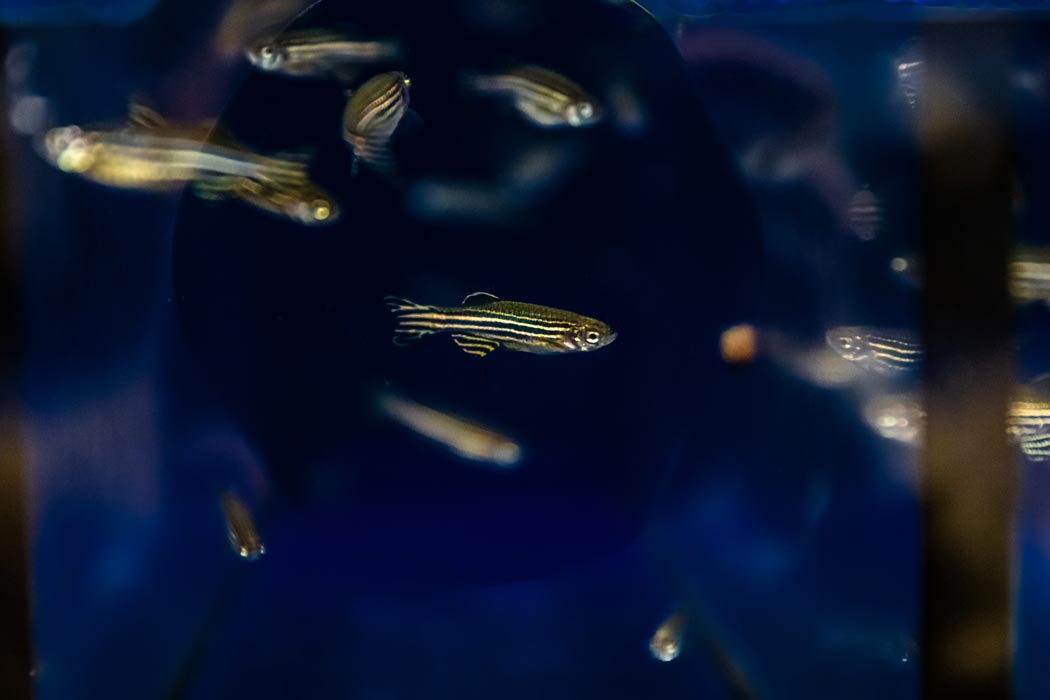
When scientists at the Institute of Science and Technology (IST) Austria looked at developing zebrafish embryos, they observed an abrupt and dramatic change: within just a few minutes, the solid-like embryonic tissue becomes fluid-like. What could cause this change and, what is its role in the further development of the embryo? In a multidisciplinary study published in the journal Cell, they found answers that could change how we look at key processes in development and disease, such as tumor metastasis.
To learn more about how a tiny bunch of cells develops into complex systems like fish, humans or animals as big as elephants, many scientists turn to the zebrafish (Danio rerio). It has a few advantages that made it one of the favorite model organisms of developmental biologists like Nicoletta Petridou, until recently postdoc at the research group of Carl-Philipp Heisenberg at the Institute of Science and Technology (IST) Austria. First, the small striped fish develop within just a few days, the embryos do so outside their mothers and are transparent – one can see every organ as it develops. While looking at zebrafish embryos a few hours after fertilization, in a previous study Nicoletta Petridou and her colleagues discovered a sudden change in the viscosity of the embryonic tissue – a measure of a tissue’s resistance to deformation: “At this early stage, the tissues forming the embryo are very rigid, but suddenly, viscosity drops by ten times and the tissue flows very quickly – it fluidizes,” the biologist explains.
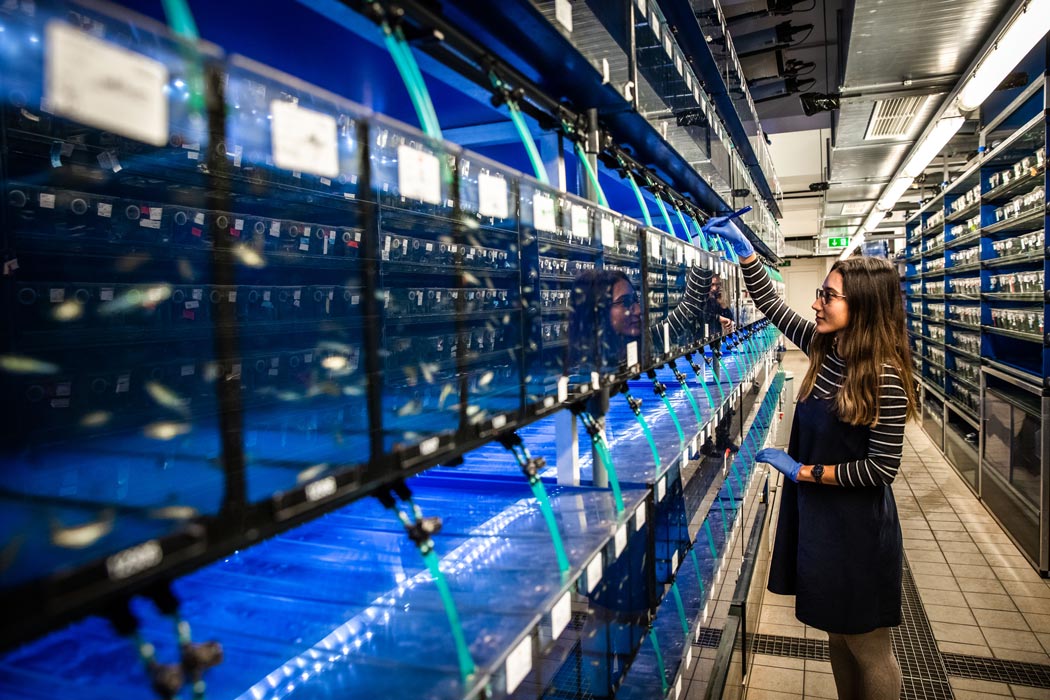
Small change – big effect
Simultaneously, the embryo starts changing its shape for the first time, thereby entering a phase called morphogenesis. In a new study, the researchers looked deeper into what is happening at the cellular level during tissue fluidization. “What we found was that before this fluidization, an individual cell is connected to four to five of its neighboring cells. At the onset of fluidization, however, it has only three to four neighbor connections left,” says Petridou. Could this small change in the cells’ connectivity really be responsible for a ten-fold difference in the tissue viscosity? “This was when we turned to physics to provide us with a framework that could explain this effect,” says the molecular and developmental biologist.
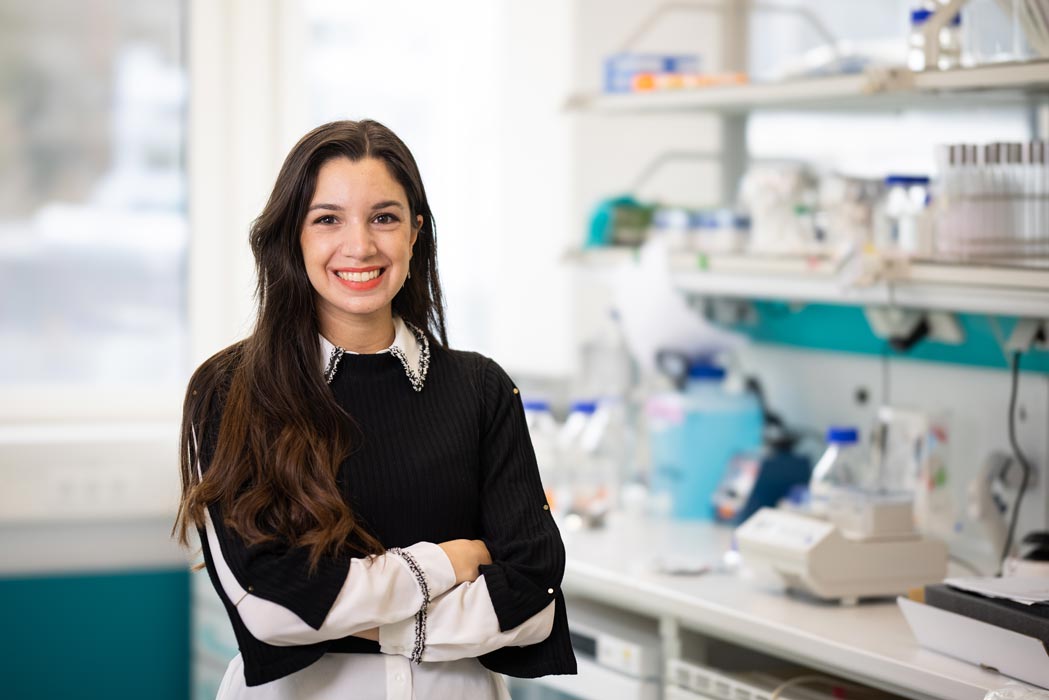
“So, Nicoletta found this massive drop in tissue viscosity at the macroscopic level that is apparently not matching what is going on at the microscopic cell connectivity level. This is a key point of physics: to link what is going on at the microscopic level with the macroscopic level,” says Bernat Corominas-Murtra, until recently part of Edouard Hannezo’s research group. Using an analogy with material science, the team realized that each cell having four connected neighbors was actually a very special threshold: physicist James Clerk Maxwell in the 19th century had already realized that structures such as bridges or networks below this connectivity level cannot be rigid anymore. This precisely matched the experimental observations of tissue fluidization occurring when cells are connected to less than four neighbors. Using this analogy, the team was able to show that the fluidization of the tissue displays features of a phase transition, which is the transition from one state of matter like solid, liquid or gas to another.
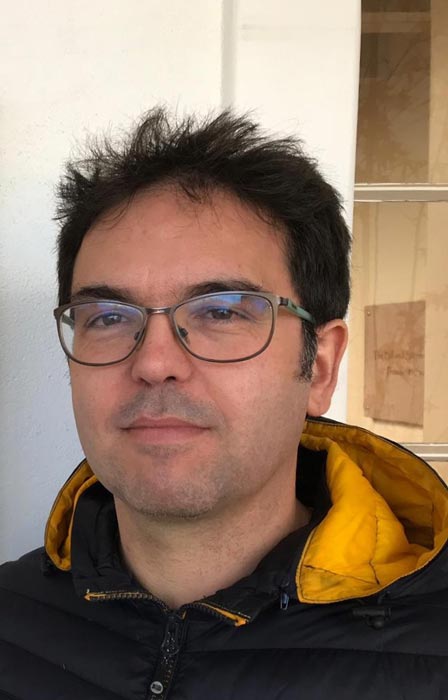
A critical point
After finding this resemblance to phase transitions, the scientists challenged their theory by manipulating the cells’ connectivity. No matter the manipulation, the critical point of connectivity was enough to explain the abrupt changes in tissue viscosity experimentally observed. Furthermore, they traced all expected features of phase transitions from the physics of non-living systems. “It is unique to be able to trace all the expected properties of a phase transition in a real, living system. Especially because the phase transition theory is made for systems with a billion components, whereas, here we are talking about a system with a few hundred components,” Corominas-Murtra explains enthusiastically.
Watch the corresponding video on YouTube
A time-lapse movie of the zebrafish embryo with an overlaid rigidity network based on rigidity percolation theory. The abrupt reduction in the size of the biggest rigid cluster (purple) marks the fluidization and phase transition at the onset of morphogenesis.
© Nicoletta Petridou, Bernat Corominas-Murtra, IST Austria
But, if the loss of only one connection per cell is enough to trigger such a significant change of the embryo’s tissue, how can an accidental change be avoided? “What was very puzzling for me was that it seems to be a very risky process for the embryo. Now that we know there is a critical point, we can ask what regulates its balance. One of the regulations we found is the timing of cell divisions that defines how tissue connectivity, and thus its viscosity, changes in space and time,” says Petridou, who has just started her own research group at the European Molecular Biology Laboratory (EMBL) in Heidelberg, Germany.
From fish embryos to tumor research
The transition is essential for the further development of the tiny fish embryo. However, it also seems to play a role in cancer growth. When a tumor metastasizes, recent studies have shown the tissue also abruptly changes from solid to liquid, which could allow cancer cells to move around more easily. “If one can trace this critical point, it opens ways to manipulate it,” says Petridou. “We don’t have the tools yet to do so, but instead of conceptually focusing on the large number of genes that could play a role in cancer growth, one could focus on this critical point that eventually causes this transition.”
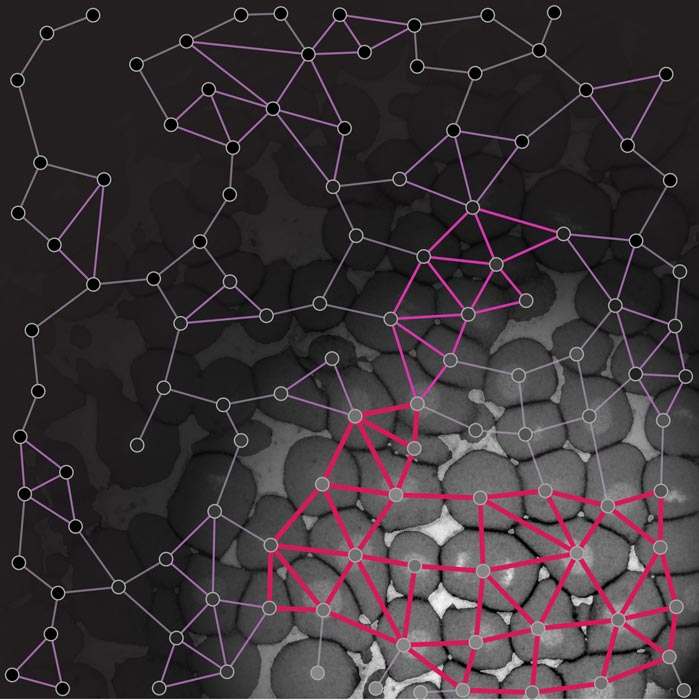
The image shows a connectivity map of the early zebrafish embryo, where the different rigid areas are highlighted (magenta). © Nicoletta Petridou, Bernat Corominas-Murtra, IST Austria
Working together with researchers from two different research groups at IST Austria proved essential for the scientists’ success. “I remember spending hours in the lab with Nicoletta trying to figure out what she was doing and the other way around. We needed to reach a common language, which takes a lot of time but is very rewarding. Multidisciplinarity is an attitude,” says Bernat Corominas-Murtra, who has recently started working as an assistant professor at the Austrian Karl-Franzens University Graz.
Publication
Nicoletta I Petridou, Bernat Corominas-Murtra, Carl-Philipp Heisenberg & Edouard Hannezo. 2021. Rigidity percolation uncovers the structural basis of embryonic tissue phase transitions. Cell. https://doi.org/10.1016/j.cell.2021.02.017
Funding information
The IST Austria project part was supported by funding from the Elise Richter Program of the Austrian Science Fund (FWF) to Nicoletta Petridou (V 736-B26), the European Research Council Advanced grant (742573) to Carl-Philipp Heisenberg, and the European Research Council Starting grant (851288) to Edouard Hannezo.